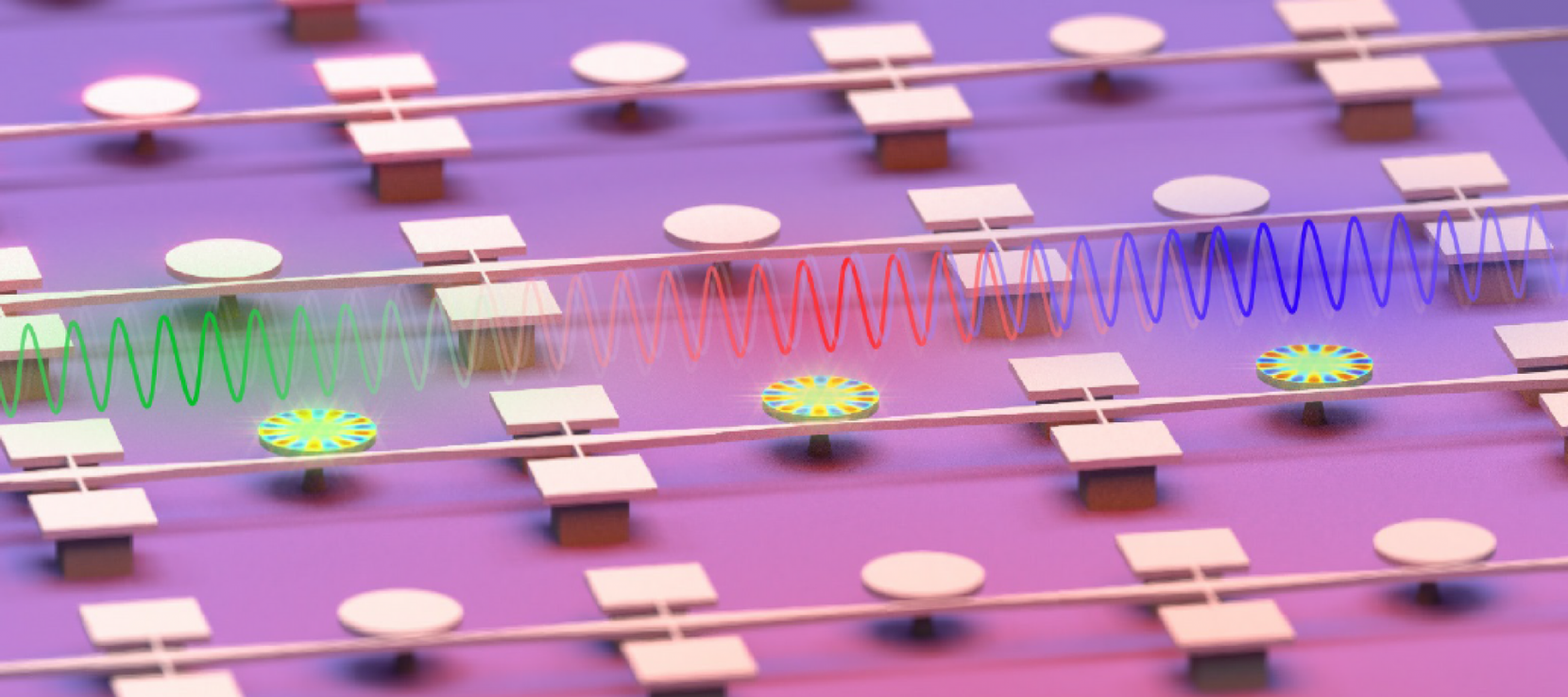
Matériaux et Phénomènes Quantiques
Présentation
The laboratory « Matériaux et Phénomènes Quantiques » (Quantum Materials and Phenomena) is a joint research unit (UMR) of University Paris Diderot and CNRS. It involves about 120 people in total with a permanent staff of 51.
The laboratory specializes in the study of frontier quantum materials and in the development of novel quantum devices. These activities rely on a large spectrum of theoretical and experimental expertise in material physics, transport and optics, and technological platforms of clean-room fabrication, spectroscopy and high-resolution electronic microscopy.
The activities of the laboratory span:
- novel materials at the nanoscale: nanocrystals, functionalized nanotubes, multiferroics, 2D materials, etc.
- novel phases of matter: quantum fluids of light, ultrastrong coupling in cavity, unconventional superconductivity, strongly correlated systems, topological phases, etc.
- nano-optical systems: optomechanics, nonlinear nanophotonics, nanoplasmonics, etc.
- quantum engineering and quantum information: quantum optoelectronic devices, quantum photonic circuits, trapped ions, hybrid organic/inorganic devices, surface and interface engineering.
Current projects of the laboratory include the development of novel probes for the investigation of quantum materials, such as time-resolved Raman spectroscopy, optomechanical atomic force microscopy, and scanning tunneling microscopy under optical excitation. Reciprocally, frontier materials are being tested as building blocks to realize novel functionalities in optomechanical sensors, nonlinear and quantum photonics devices, or in cavity embedded transport experiments.
[hal-01987572] Imaging the symmetry breaking of molecular orbitals in single-wall carbon nanotubes
Date: 21 Jan 2019 - 11:19
Desc: Carbon nanotubes have attracted considerable interest for their unique electronic properties. They are fascinating candidates for fundamental studies of one dimensional materials as well as for future molecular electronics applications. The molecular orbitals of nanotubes are of particular importance as they govern the transport properties and the chemical reactivity of the system. Here, we show for the first time a complete experimental investigation of molecular orbitals of single wall carbon nanotubes using atomically resolved scanning tunneling spectroscopy. Local conductance measurements show spectacular carbon-carbon bond asymmetry at the Van Hove singularities for both semiconducting and metallic tubes, demonstrating the symmetry breaking of molecular orbitals in nanotubes. Whatever the tube, only two types of complementary orbitals are alternatively observed. An analytical tight-binding model describing the interference patterns of pi orbitals confirmed by ab initio calculations, perfectly reproduces the experimental results.
[hal-02407634] Optomechanics: a key towards next-generation experiments in atomic force microscopy?
Date: 12 Dec 2019 - 15:49
Desc: Atomic Force Microscopy (AFM) is a versatile and ubiquitous technique based on a resonating tip probe that interacts with the sample to be analysed in terms of topography and mechanical properties. Next-generation investigations in molecular biophysics like protein folding/unfolding, receptor-ligand interaction and molecular diffusion on cell membrane, require tracking molecular forces at the nanosecond timescale in a non-perturbative manner [1]. Conventional AFM probes, made of micro-cantilevers vibrating in the MHz range with nanometer amplitudes and combined with an optical deflection detection system, are the current bottleneck to reach the required performances. Both aspects, a very low and non-perturbative vibration amplitude, time resolution, and measurement bandwidth, are impacted by the same chief parameter: the frequency f of the probe mechanical resonator. While a higher frequency unlocks the bandwidth and the time resolution, it also sets the Brownian motion low enough to provide exquisite signal-to-noise ratio and force resolution even for vibration amplitude in the picometer range, i.e. much lower than the molecular dimensions. Recent advances in optomechanical devices technology allow tackling the challenge by offering resonators at very high frequencies greater than the GHz and unprecedented motion sensitivity below 10-17 m.Hz-0.5. The talk will present our recent developments introducing a fully-optically operated resonating optomechanical AFM probe above 100 MHz of frequency, 2 decades above the fastest commercial cantilever probes, while Brownian motion 4 orders below [2]. Based on a silicon technology and operated at 1.55 µm wavelength, the probe shown in Fig. 1 demonstrates high-speed sensing of mechanical interactions with a sub-picometer resonantly driven motion, breaking open current locks for faster and finer force spectroscopy at the molecular level. Figure 1. Scanning electron microscopy image of the optomechanical atomic force microscopy probe. The ring-shaped whispering gallery mode resonator is 20 µm in diameter. A 4 µm-long tip apex protrudes from the ring, aiming at sensing near-field forces when interacting with a surface.[1] H. Yu et al., Science, 355, 945-950, (2017)[2] P.E. Allain et al., arXiv:1810.06209, (2018)
[hal-00548728] Microwave modulation of terahertz quantum cascade lasers : a transmission-line approach
Date: 30 May 2022 - 17:04
Desc: We report on microwave impedance measurements of metal-metal ridge-waveguide terahertz quantum cascade lasers. Experimental data, recorded at 4 K in the 100 MHz–55 GHz range, are well reproduced by distributed-parameter transmission-line simulations, showing that the modulation cutoff is limited by the propagation losses that increase for higher microwave frequencies, yielding a 3 dB modulation bandwidth of ∼70 GHz for a 1 mm-long ridge. By using a shunt-stub matching we demonstrate amplitude modulation of a 2.3 THz QCL up to 24 GHz.
[hal-00356949] Metal-metal terahertz quantum cascade laser with micro-transverse-electromagnetic-horn antenna
Date: 29 Jan 2009 - 09:50
Desc: A 2.8 THz metal-metal quantum cascade laser operating with a microtransverse-electromagnetic-horn antenna has been demonstrated. By comparing the far-field radiation pattern to a standard ridge cavity with a cleaved facet, a striking improvement of the directionality is observed. The effectiveness of the horn antenna is critically dependent on the control of the lateral modes in the laser cavity.
[hal-01908683] Optomechanical Resonating Probe for Very High Speed Sensing of Atomic Forces
Date: 21 Jul 2022 - 13:06
Desc: Atomic force spectroscopy and microscopy (AFM) are invaluable tools to characterize nanostructures and biological systems. Most experiments, including state-of-the-art images of molecular bonds, are achieved by driving probes at their mechanical resonance. This resonance reaches the MHz for the fastest AFM micro-cantilevers, with typical motion amplitude of a few nanometres. Next-generation investigations of molecular scale dynamics, including faster force imaging and higher-resolution spectroscopy of dissipative interactions, require more bandwidth and vibration amplitudes below interatomic distance, for non-perturbative short-range tip-matter interactions. Probe frequency is a key parameter to improve bandwidth while reducing Brownian motion, allowing large signal-to-noise for exquisite resolution. Optomechanical resonators reach motion detection at 10^(-18) m.(Hz)^(-1/2), while coupling light to bulk vibration modes whose frequencies largely surpass those of cantilevers. Here we introduce an optically operated resonating optomechanical atomic force probe of frequency 2 decades above the fastest functional AFM cantilevers while Brownian motion is 4 orders below. Based on a Silicon-On-Insulator technology, the probe demonstrates high-speed sensing of contact and non-contact interactions with sub-picometre driven motion, breaking open current locks for faster and finer atomic force spectroscopy.
Autres contacts
Université Paris Diderot - Paris 7
U.F.R. Physique
Bâtiment Condorcet
10, rue Alice Domon et Léonie Duquet
75205 PARIS CEDEX 13